By Michael Niedzinski, Constellium.
During the past 60 years the National Aeronautics and Space Administration (NASA) charted the western world’s course for the exploration of outer space. NASA managed and funded programs such as Gemini, Apollo, Skylab, and the International Space Station, which is supported by Space Shuttle missions and more recently by the Space Launch System (SLS). The most significant current program is the SLS (Figure 1), which is aimed at exploration of the Moon and nearby planets. The SLS launch systems will be based on both the cryogenic fuel/oxidizer and the solid fuel booster architectures. It will be a two-stage rocket, using liquid oxygen/hydrogen for propulsion. The Boeing Corporation is the primary contractor responsible for integration of all SLS systems and their eventual launch in 2020. The current strategy is to have both cargo and crew variants capable of hoisting up to 130 tons into various orbits or into planetary trajectory. Some variants will hoist cargo for eventual missions to either the Moon or Lagrangian points (the points near two large bodies in orbit, such as Earth and the Moon, where a smaller object will maintain its position relative to the large orbiting bodies), which could be used as staging sites for other longer missions. Up to seven astronauts will be delivered to these destinations using the Orion Crew Module that was developed and built by Lockheed Martin Corporation.
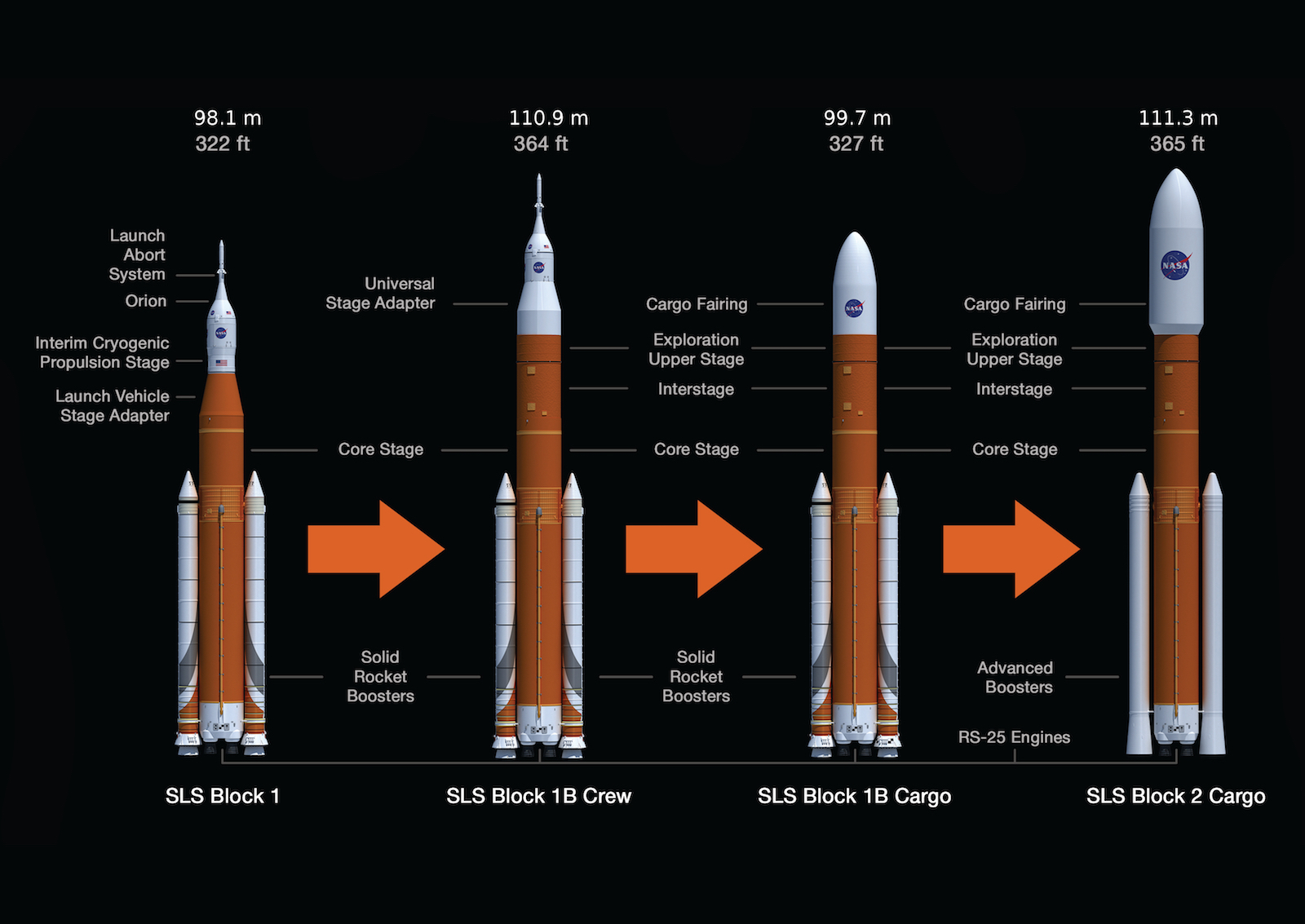
Concurrent to the SLS activity, NASA has been encouraging and funding private industry to develop launch systems that can provide resupply capability to the International Space Station. This was driven by the need to fill the vacuum created by premature cancellation of the Space Shuttle program. The expectation was that this stimulus would lead to competition and provide lower cost and hopefully more efficient means for space exploration. Consequently, during the last 15 years, there has been an unprecedented growth in interest and investment in the exploration of space by non-government entities. Elon Musk’s SpaceX started this trend with the Falcon 1, Falcon 9, and Falcon Heavy rockets, which initially acted as cargo carriers to the International Space Station and satellite launch platforms. More recently, these SpaceX programs have shifted focus to exploration of the nearby planets. The company’s fully reusable BFR/Starship is being designed for exploration and colonization of Mars with its first flight to the Moon planned for 2023.
Other private companies have also entered the sector. Jeff Bezos’ Blue Origin is building the New Glenn with launch objectives that are likely very similar to SpaceX. This includes a three-stage variant rocket that will also likely have a capability to explore the Moon. United Launch Alliance is preparing their new low cost rocket, called Vulcan, to replace the aging Atlas V and Delta IV. Northrop Grumman acquired Orbital Sciences–ATK and is moving forward with a three stage Omega space launcher. A number of smaller organizations are demonstrating capability for suborbital flights and tourist business, providing short (under 60 minutes) excursions up to about 62 miles altitude in order to allow customers to experience weightlessness and see the Earth’s curvature. Several of the new U.S. launch platforms are shown in Figure 2, with precursors (such as Saturn V from the Apollo program and the Russian Soyuz) provided for comparison.
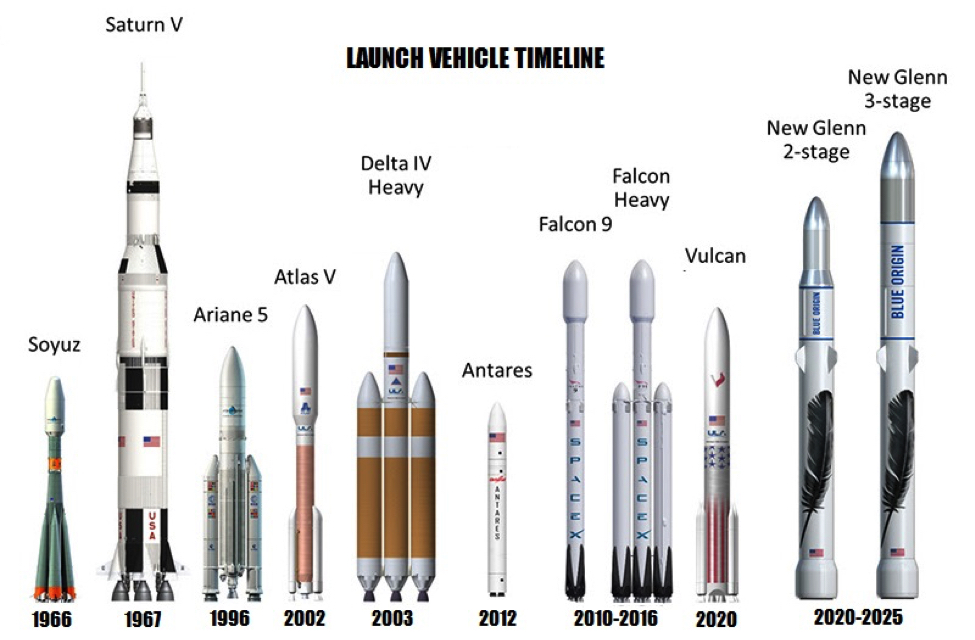
Outside the U.S., a very reliable Ariane 5 will be replaced by Ariane 6 which is being built by ArianeGroup under the authority of the European Space Agency, with a first test flight scheduled for 2020. Ariane 6 is being designed to compete with the low cost Falcon 9 program. South Korea, India, and Japan have also been very successful in both launches and prototyping of new vehicles.
Space Launch System Design
Basically space launchers (rockets) consist of fuel/oxidizer tanks, complex pumps and fuel delivery systems, guidance and control systems, a rocket engine, and the payload module. Certain designs call for usage of side mounted solid rocket boosters that provide the extra boost during the early stages of the liftoff. Two types of design concepts have been adopted for the cryogenic fuel tanks: monolithic integrally stiffened panels or sheet metal stiffened with longitudinal and radial extrusions to provide requisite rigidity. The first concept utilizes thick and wide plate, which is machined to produce a membrane about 0.125 inches thick with stiffeners 1-3 inches tall. These panels are formed to produce curved sections, aged to a final temper, and finally joined together to form a cylinder.
In the second concept, smaller sheet metal panels ranging in thickness from 0.25–0.50 inches that are 70-90 inches wide and up to 300 inches long are formed into sections and welded to produce cylinders. Rigidity and strength is obtained through the friction stir welding (FSW) of longitudinal stringers to the inside of the cylinder and then mechanically fastening radial stiffeners.
Two or more of these cylinders—produced using either method of construction—are stacked together to form the fuel tank, which will hold liquid hydrogen or kerosene and an oxidizer (normally liquid oxygen). The cylinders are capped with spun-formed domes using FSW. This article will concentrate on systems built using the monolithic integrally stiffened panel construction method.
Use of Aluminum in Space Launchers
The cost of launching cargo into space is very high, thus the vast majority of both old and new rockets rely on aluminum solutions due to the material’s light weight and cost. Metallic materials for space launch vehicles and crew modules have very specific requirements due to the extreme environmental and operational stresses that occur when the rocket is in use. For example, the propellant and oxidizer tanks of the liquid fueled rocket are pressurized, requiring high tensile strength as a prime deliverable. Likewise, individual stages of the space launchers are stacked on top of one another; thus, high resistance to compressive loading is required. A high tensile and compressive modulus are needed to minimize flexure during turbulence of initial stages of the launch. Furthermore, the structure of the launcher must have the lowest possible density while maintaining strength in order to maximize the payload and orbit options.
A typical oxidizer for the current generation of rockets is cryogenic oxygen, which is maintained at about -200°C and propellants range from cryogenic hydrogen at -250°C to liquid natural gas (-160°C) or kerosene (-47°C). Therefore, the ability to maintain good fracture toughness at these temperatures is essential. Space launch designers require that the metallic tankage solutions exhibit 10-30% improvement in fracture toughness when tanks are chilled to the cryogenic range. Due to the extreme temperature variations experienced by the launcher (cryogenic liquid on the inside and ambient air on the opposite side, separated by thermal protection system and metallic membrane 0.125-0.250 inches thick), predictable coefficients of thermal expansion and thermal conductivity are needed. A number of 2xxx alloys meet these requirements, while a number of 7xxx alloys are embrittled by exposure to these extremely low temperatures.
In addition, fuel tanks are stored for significant periods of time in a tensile stress inducing horizontal position in coastal areas where the corrosive (NaCl) environment creates conditions conducive to stress corrosion cracking (SCC). This makes these products susceptible to induced corrosion. Thus high resistance to SCC is mandatory. To ensure ease of fabrication, good formability and machinability in T3X temper coupled with good weldability (current trend is to use FSW exclusively) is necessary. Finally, Tier 2 suppliers and machine shops must be comfortable with the processing of these materials.
Development of Aluminum-Lithium Alloys
Originally (with few exceptions) all past designs for cryogenic fuel tanks and all the structural space launcher components relied on alloys such as 2014, 2219, 7050, and 7075—which are 40-70 years old. While these alloys are proven performers, they represent a very conservative (low payload/low orbit) solution. In order to maximize the payload for resupply missions or geostationary/geosynchronous orbit, Constellium developed several ultra-high performance aluminum-lithium alloys. Initially operating as Reynolds Metals, the company in cooperation with Lockheed Martin and NASA developed a 2195 (AL-Li-Cu-Zr) alloy. In 1994, this alloy was selected for the cryogenic sections of the Super Light Weight External Tank (SLWT), which is an integral component of the early Space Shuttle launch systems. The 2195 alloy replaced the legacy 2219 aluminum-copper alloy, providing over 6,000 lbs in weight savings that translated into payload improvements due to its higher strength/modulus, reduction of SCC, and 5% lower density. Forty Space Shuttle missions utilized the SLWT to transfer large components to the International Space Station, which would not have been possible without the reengineered alloy.
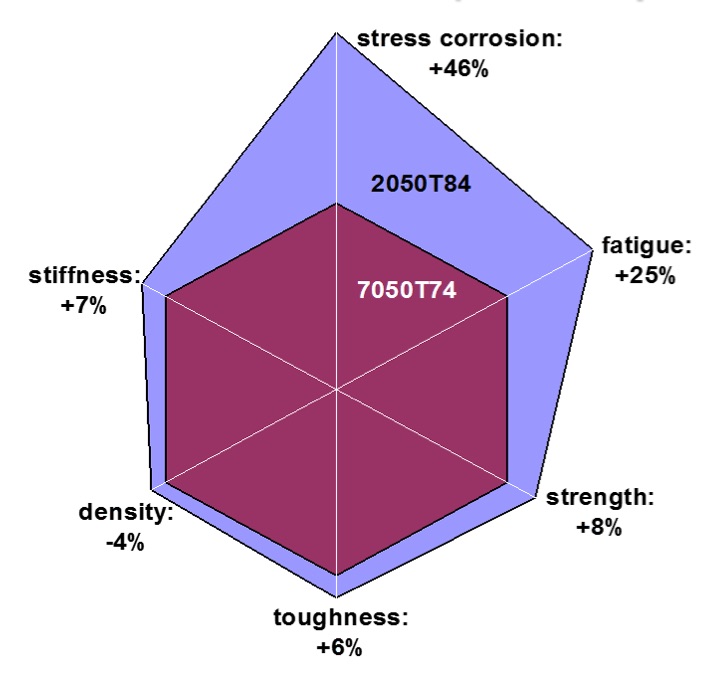
While high strength alloy 2195 is an excellent choice for structures comprised of plate that does not exceed 2.25 inches (57 mm) in thickness, it was not recommended for structures that required plate in greater thicknesses (based on lack of relevant data). In 2004, the company (operating as Rio Tinto Alcan) developed a 2050 alloy in order to address the requirements of structures with larger thicknesses in the range of 2.25-6.50 inches (57-160 mm). As an evolution of the previously designed 2195, alloy 2050 was engineered to match and/or exceed the tensile and fracture properties of legacy alloy 7050-T7451 while providing a 4% density reduction and up to 5% elastic modulus improvement (Figure 3). Initially targeted at thick frames, bulkheads, and wing ribs of commercial and military aircraft up to 6.5 inches thick, the 2050 alloy was ultimately evaluated for cryogenic tank applications for space launch vehicles based on its excellent fracture toughness and stability at cryogenic temperatures.

When Alcan Engineered Products was spun off from Rio Tinto Alcan, the company changed its name to Constellium and introduced the Airware® brand name. Both the 2195 and 2050 alloys joined a growing family of Airware alloys designed specifically to meet the needs of the aerospace and defense sectors. Specific characteristics of these alloys, such as 2195 and 2050 are based on a unique microstructure, which consists of a high density of very fine platelet particles identified as T1 (Al2CuLi). These fine precipitates anchor and slow down movement of dislocations yielding a high strength and other characteristics. In fact, T1 (Figure 4) is considered to be the most efficient strengthening precipitate phase known for aluminum, providing the highest increase in strength per volume fraction.
Over the past ten years, Airware alloy 2050 joined 2195 as a preferred plate material for both pressurized and non-pressurized structural components used in space launchers and crew modules requiring a thickness range of 0.500–6.500 inches. Alloy 2050 is produced per AMS 4413 (2050-T84) and AMS 4372 (2050-T34/T82) guidelines and a full set of allowables for the alloy was developed and included in the Metallic Materials Properties Development and Standardization (MMPDS) handbook, considered a primary reference for designers of aircraft and space launch systems.

Alloy 2050 offers comparable improvements in strength and modulus with an A rating in the SCC area, while also expanding the thickness range to match the applicable gauge ranges found in legacy alloys 2219 and 7050. Multiple users of the alloy have noted significant improvements in cryogenic toughness, a key requirement. Compared to legacy 2219 (Figure 5), alloy 2050 exhibits about 30% plus higher yield strength, 5% higher compressive and tensile modulus, and a 5% lower density. This permits a design that is significantly stronger with a higher rigidity, providing a 5% weight reduction for drop-in replacement of cryogenic tank components. When structures are also reengineered to use thinner stiffeners with wider pocket spacing, the alloy can demonstrate up to a 15% weight reduction.
Another key parameter for cryogenic tanks is freedom from embrittlement or enhancement when exposed to cryo temperatures. Alloy 2050 shows significant improvement in both static (tensile strength) and dynamic (fracture toughness) properties at both liquid oxygen and hydrogen temperatures. Aluminum plates of various thicknesses of 5 and 6.5 inches were tested at both liquid oxygen and hydrogen temperatures and compared against room temperature behavior (Figure 6).
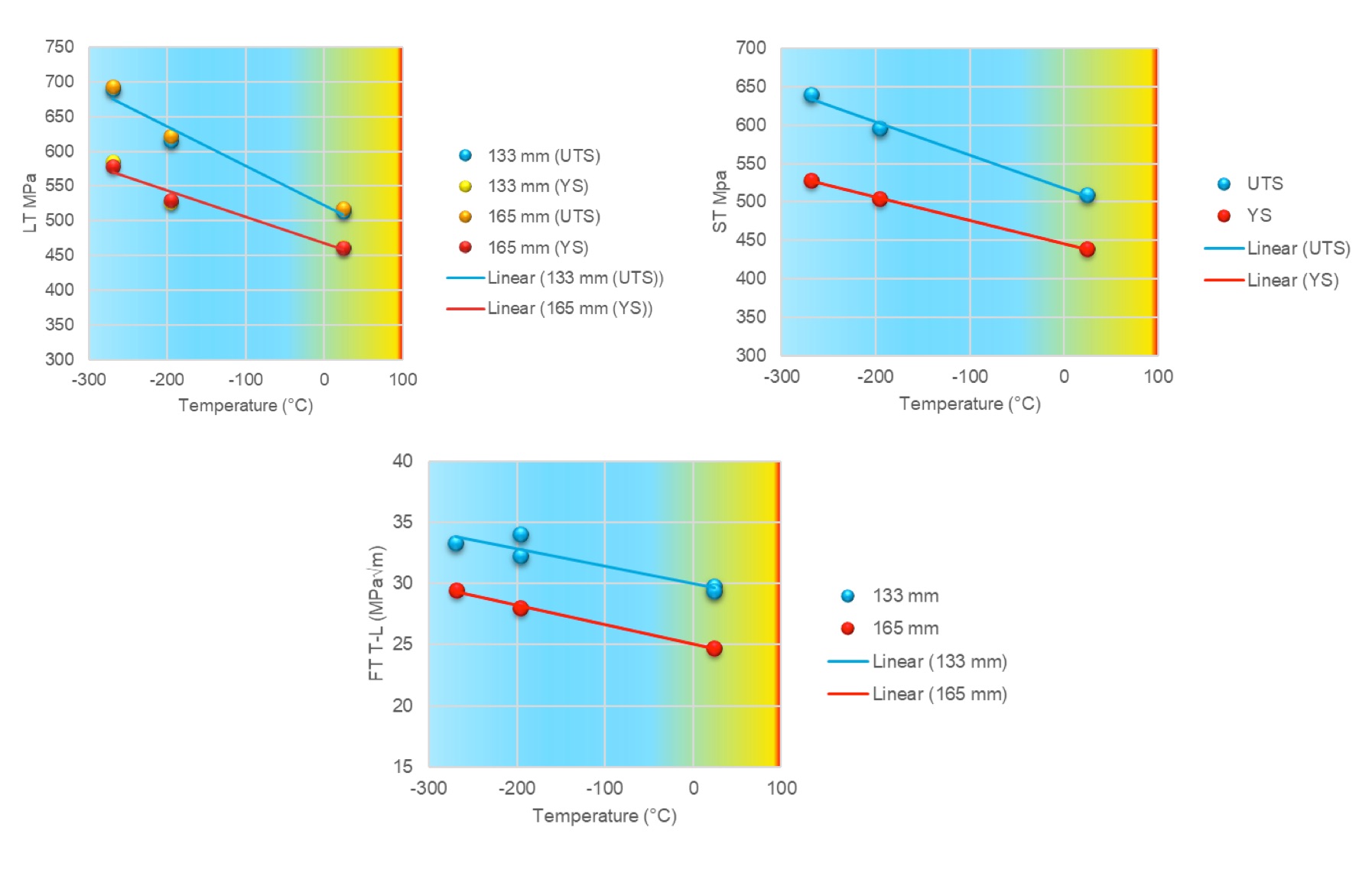
Addressing the challenge that comes with long-term storage of tanks either at Cape Canaveral or other marine locations, alloy 2050 provides enhanced corrosion resistance. As a part of its material acceptance process, the alloy is routinely tested per requirements of AMS 4413 and ASTM G47 (susceptibility to SCC), in which materials are tested in the short transverse direction at a 45 ksi minimum threshold stress for 30 days. For comparison, legacy alloy 2219 has a 37 ksi minimum for 30 days. At the request of the space launch system manufacturers, Constellium tested 2050 using more extreme threshold stress conditions over longer exposure periods. In various gauges, 2050 was able to withstand harsher test conditions than specified in AMS 4413.
Another attribute that is rapidly gaining importance for materials used in cryogenic tanks is the ability to resist softening during exposure to elevated temperatures. The current trend is to design launchers with reusable components, which presents challenges since post launch reentry of the core or second stage rocket sequence generates high surface temperatures. Even though reentry time is measured in minutes, it is important that tensile, fracture, and corrosion characteristics are not altered during this high temperature period, thus permitting the reuse of the component. Similarly, crew modules or space habitats may be exposed to intense heat from the sun while in orbit or at a Lagrangian point. Alloy 2050 is very stable up to 350°F, when multiple cycles that last 3-10 minutes or long term exposure while in orbit are considered. Post exposure tensile strength for various time temperature cycles is shown in Figure 7.

Beyond the requirements for the superior mechanical properties and corrosion resistance mentioned, any alloy candidate must exhibit two other important characteristics—good formability and machinability. Alloy 2050 is readily formable and bendable in the T34 temper, which is used for multiple applications in the space program. The T34 temper provides an aggressive bend radii as low as R/t =3 (R/t is a ratio of radius of curvature to thickness of the material undergoing a three-point bending process). Bending via bump forming or break forming is equivalent to legacy alloy 2219-T37. The alloy’s excellent ductility (elongation in excess of 20%) in T34 temper (solution heat treated and cold worked) permits production of barrel and cone sections through conventional forming processes, such as roll and bump forming, on plates up to 150 mm thick.
Alloy 2050 machines very readily in both T34 and final T84 tempers, using a full range of speeds on 3, 4, and 5 axis milling centers with either horizontal or vertical tables. A wide range of parts and parameters are used with aluminum input plate ranging from 100 to 12,000 lbs. High part complexity is possible with single side machining, pocketing, and asymmetry. The lubrication methodology for machining 2050 includes both flood cooling and micro-lubrication with a speed range of 15,000 to 40,000 rpm. Tool wear, chip formation, and distortion is similar to that observed on other high strength conventional aluminum alloys, such as 7050 and 2219. As with other high strength alloys, machining centers working with 2050 need to develop a metal removal strategy to ensure freedom from distortion.
In addition, 2050 can be readily joined via the FSW process. Joining of dissimilar base materials such as 2050 and legacy 2219 indicated a nugget strength higher than base 2219 plate. When compared to 7050-T7451, alloy 2050-T84 also exhibits an improvement in standard fatigue and fatigue crack growth, as well as a significant improvement in spectrum fatigue performance. This may have high importance because of the current emphasis on multi-use launch vehicles.
All of the attributes noted here allow for fabrication of large 2050 aluminum panels with a nominal size of 100-150 inches wide and up to 500 inches long. Plates range in thickness from 1.00-4.00 inches. A preferred method for designers is to use 2050 plate in the highly formable T34 temper with elongation exceeding 20%. Constellium developed the AMS 4372 specification, covering 2050-T34 and -T82 aging capability response, which can support T84 in AMS 4413 in the final formed, machined, and aged configuration.
The manufacturing process to produce a typical multi-stage liquid fueled launch system cylinder (Figure 8) involves the machining of 2050-T34 plates to create stiffened panels, which are then break-formed and artificially aged to T8 condition. The thermal treatment attains both requisite mechanical properties and a controlled reduction of the residual stress that developed during the machining and break forming operations. An example of a fabricated 2050-T84 cylinder panel prior to joining is shown in Figure 9. Tier 2 aerospace component fabricators based in the U.S., such as AMRO in Los Angeles, CA, have developed methods to machine, bump form, and artificially age 2050 panels into multiple configurations, such as iso-grid and ortho-grid geometries. Component integrators use the longitudinal FSW process to join panels to form a cylinder. Multiple cylinders (2-5) are then stacked and joined using radial FSW methodology, followed by spun domes that are joined to complete the tank.
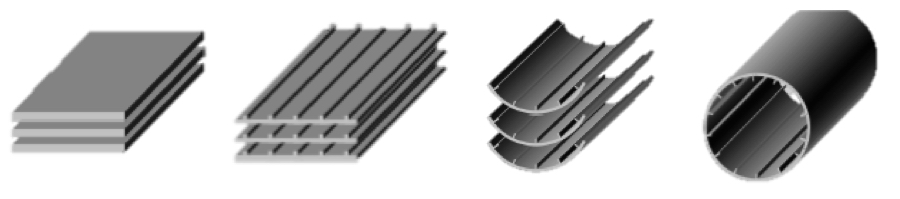
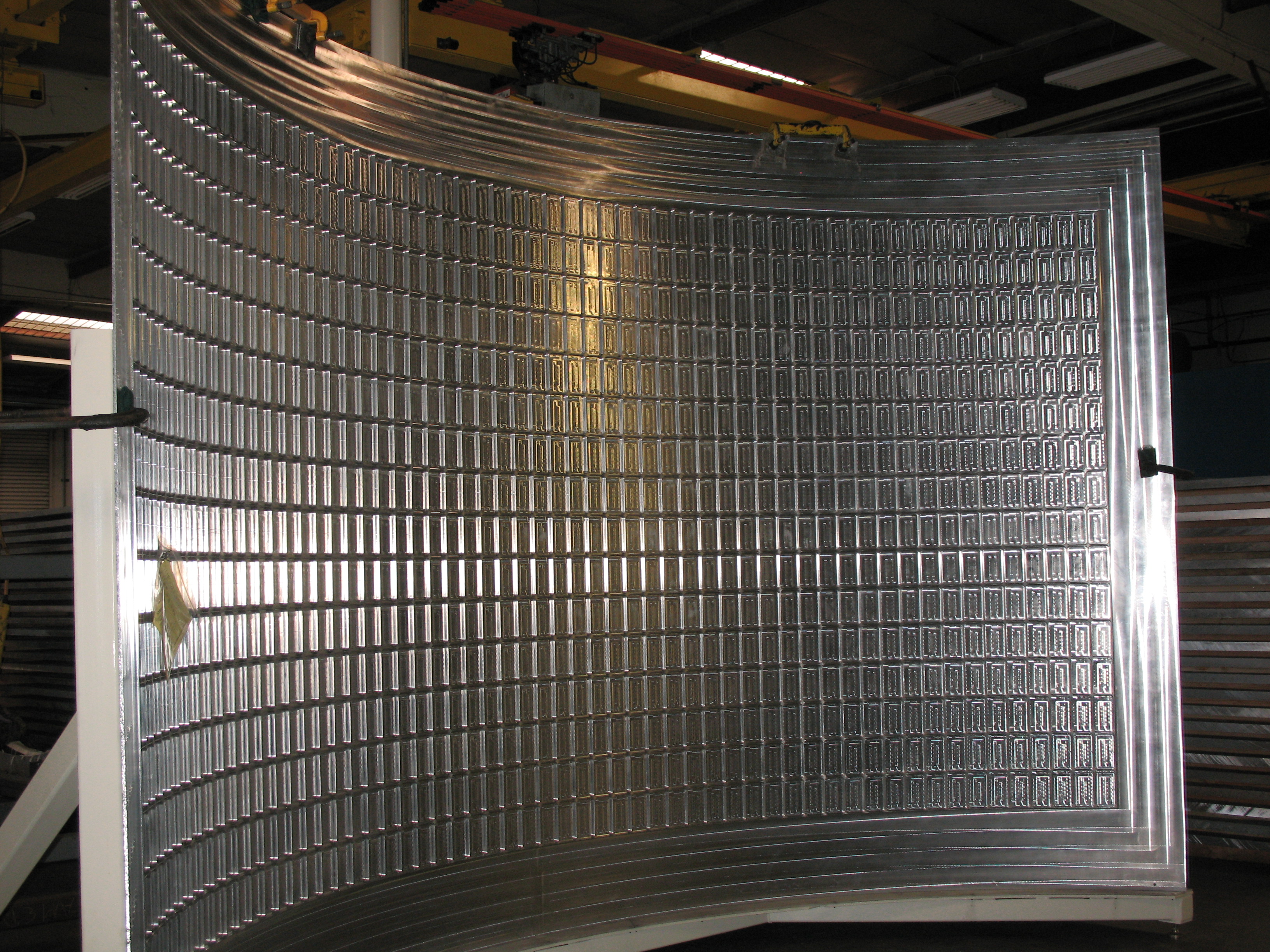
FSW Joining
In the past, individual legacy 2219 and 2014 panels were joined using metal or tungsten inert gas (MIG or TIG) welding methods. This process resulted in about a 50% knockdown factor (percentage strength loss when compared to the base material) for the weld area. This high knockdown necessitated usage of thick weld lands, adding to the weight of the vehicle. Unlike alloys 2219 and 2014, alloys 2050, 2195, and other conventional 2xxx alloys that contain magnesium are not readily fusion weldable. Porosity in the weld nugget area and oxidation of molten metal pool are the major issues, which can be partially overcome by a very tedious variable polarity plasma arc (VPPA) welding process. VPPA requires two side shielding of the weld with inert gases, such as argon, and yields the same 50% knockdown factor.
To overcome this constraint the FSW solid state welding process was developed in order to facilitate the joining of individual 2195 and/or 2050 panels for the production of cylinders for cryogenic tanks. The process was pioneered about 20 years ago during the manufacture of the SLWT tank for Space Shuttle missions, when Airware 2195 replaced legacy 2219. The FSW process reduces knockdown factors to about 20-30%. Another big benefit is reduction of the weld land height, which further reduces the weight of the component. The aluminum can be joined in both T34 and T84 condition. Experience shows that higher weld area properties can be achieved by joining in the T34 temper and then subsequently aging the material to final T84 condition. Certain structures, due to their size, require joining in the T84 condition. About 8% higher tensile properties are possible through selection of the T34 joining/post weld aging method.
Several years ago, Constellium worked with Institute de Soudure to perform a study aimed at characterization of the FSW joints to develop a better understanding of the final product. In order to demonstrate the stability of long longitudinal welds typical for 2050 aluminum panels, a 400 inch long weld was produced. Specimens representing base metal, heat affected zone, and the weld nugget were excised from the weld area. The test portfolio included tensile properties, fracture toughness, fatigue, fatigue crack growth rate, and SCC resistance. The FSW equipment, test coupons location, and morphology of the weld is shown Figure 10 while test the coupon cut up plan is shown in Figure 11.
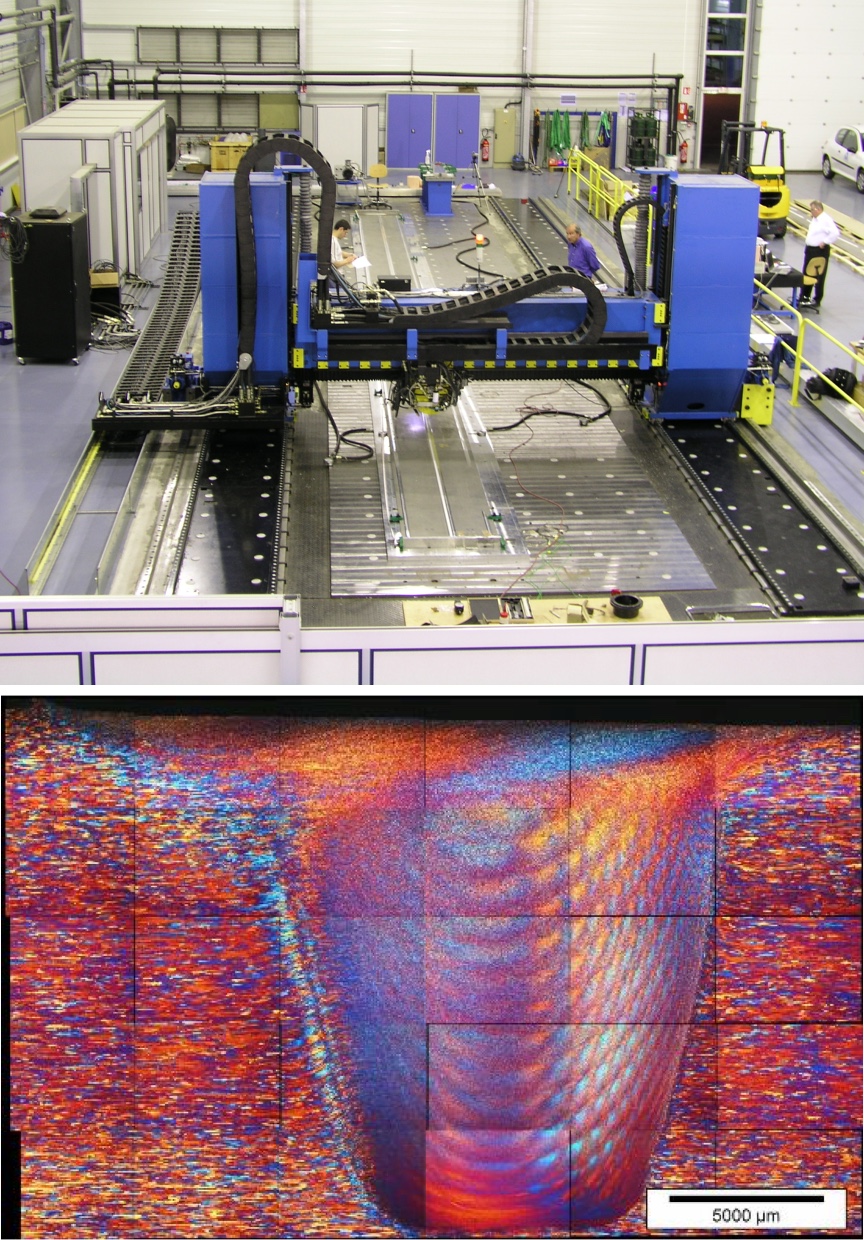
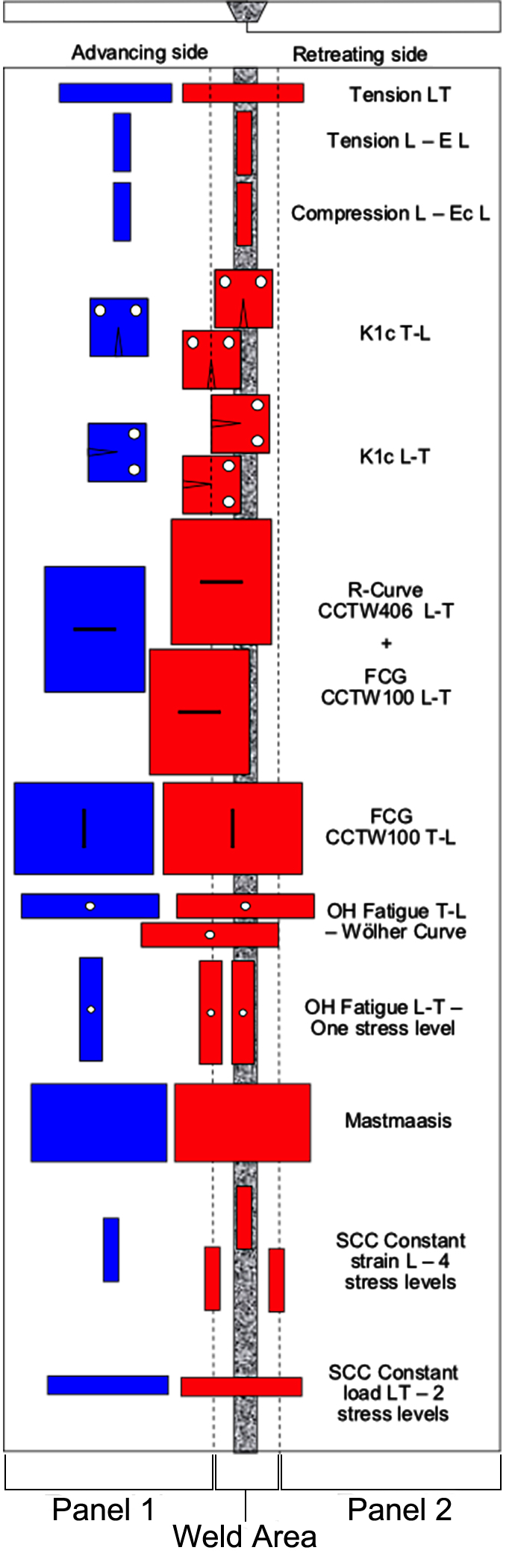
The test results are summarized hereafter. Extensive static, fracture, and damage tolerance characterization of the nugget and heat affected zone showed that the static knock-down factor was confirmed to be limited to a 20% reduction of tensile properties in the weld nugget when compared to base material. A slight decrease in the precision tensile (E) and compressive (Ec) modulus was noted. Fracture toughness was improved while corrosion resistance (according to stress corrosion and Mastmaasis salt spray tests) are not degraded versus the base metal. Fatigue crack growth rate (FCGR) is decreased in the heat-affected zone but is improved/increased in the nugget area. Overall, the number of cycles across a weld is larger than for the base metal. Similarly, open hole fatigue shows improvement versus the base metal. Thus, the feasibility of long FSW weldments in 2050 alloy was demonstrated through several trials, which were thought to show world firsts in welding time, bringing this technology to the readiness level required for industrial use for long aero-structures. FSW of 2050 alloy, whether for long or more conventional size aero-structures, is thus proved to be a reliable technology, permitting a decrease in the buy-to-fly ratios and in the cost of these structures.
Designing Crew Modules
SpaceX, Lockheed Martin, and Boeing have either flown or are finalizing design of their crew and cargo vehicles named Dragon, Orion, and Starliner, respectively. Crew and cargo vehicles utilize monolithic heavily machined components requiring thick plates with low residual stresses to minimize distortion during the machining process. These components are not considered to be cryogenic critical, thus a variety of aluminum alloys are commonly implemented, including 7050 and 2219. A number of the smaller components on some of these vehicles were converted from legacy 2219 to 2050 to save weight.
Currently, a trade study and prototyping program is being conducted by Constellium, AMRO, and Westmoreland Mechanical Testing and Research, Inc. (an independent lab in Youngstown, PA). The study aims to demonstrate the value of 2050 for these kinds of structural components used in the crew modules that will carry astronauts or cargo into space. Feasibility of converting large structural components was evaluated through the development of a partial cone component for a crew module, which required very complex forming and extensive machining to achieve final configuration. This demonstrator component required complex forming and machining of plates in excess of 6 inches in thickness. For this study, Constellium produced 2050-T34 plate with a nominal weight of over 11,000 lbs to demonstrate process feasibility. The plate, processed at AMRO, was sawed into a forming blank and then bump formed into a machining blank. Both surfaces of the part were machined to achieve final geometry. The fabrication sequence is shown in Figure 12. The finished part was artificially aged and samples were extracted from the porthole regions. The material was tested at Westmoreland Mechanical Testing.

Using the samples excised from the porthole, excellent mechanical properties, far exceeding legacy 2219 were observed on the finished component. Very uniform properties were reported for the four typical tensile testing directions: Longitudinal, Long Transverse, 45, and Short Transverse at both T/2 and T/4 test planes (Tables I and II). A high level of isotropy was observed in the four directions tested, which each represent a mid and quarter thickness of the original 6 inch thick plane. Processing characteristics, specifically ease of forming and high stability during the machining of 2050 plate were cited by AMRO. This data is being reviewed by potential users to assess usability for their specific applications. On this particular component, successful conversion from legacy 2219 to 2050 would yield a 190 lb weight reduction through both density differential and redesign.


Summary
Space launch systems and space travel require materials that push the requirements envelope. Sophisticated aluminum-lithium alloys, such as Airware 2195 and 2050, permit a stronger yet significantly lighter architecture—allowing more payload, higher orbit, or both. Alloy 2050 in either a highly formable and ductile T34 or T84 temper is being readily adopted for both cryogenic tanks and crew modules. The alloy has also been baselined for the second (upper) stages of two major space launch programs. Trade studies indicate that core stage is also a suitable candidate for conversion to 2050 from legacy 2219. Numerous other noncryogenic applications, such as intertanks, adapters, and engine sections are currently being studied for conversion. In some cases, hybrid designs with both 2195 and 2050 applications are being implemented on the same launch vehicle. In these hybrid designs, the upper stages that require lighter gauge plate favor higher strength 2195, while components of the core stage call for 3.000-4.000 inch thick 2050 plate. Similarly, a significant amount of 2050 plate with complex shapes has been successfully tested and prototyped for the Orion Crew Module. Through its ongoing effort to provide cutting edge materials at its Ravenswood, WV, and Issoire, France facilities and C-TEC Research and Development Center near Gernoble, France, Constellium has an opportunity to play a very important role in human exploration of our planetary system.
Michael Niedzinski MS/MBA serves as director of Standardization and CAE group leader Space & Defense at Constellium. He has been involved with space programs such as the space shuttle, Space Launch System, SpaceX, Blue Origin, ULA, Ariane 6, and Orion since 1994. He helped to develop and commercialize AIRWARE alloys 2195, 2098, 2050, and 2297 for space launch and defense initiatives over the past 20 years. He has been granted three patents related to new aluminum alloys and has received NASA’s Sustained Effort Award (1996) and the U.S. Army’s Defense Manufacturing Achievement Award (2017). Niedzinski is a member of ASM, MMPDS, ASTM, AMS, Aluminum Association, and NADCAP.
Editor’s Note: This article first appeared in the February 2019 issue of Light Metal Age. To receive the current issue, please subscribe.